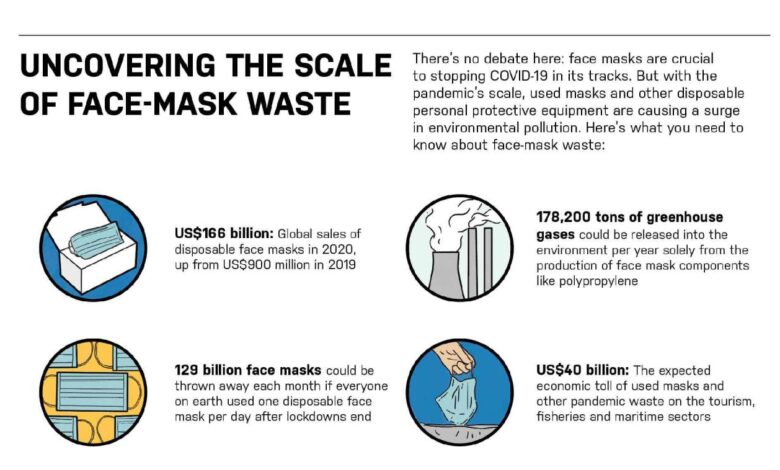
The simple science behind why masks work is fascinating. This post dives into the detailed mechanics of how masks prevent the spread of respiratory droplets, from the basic physical barriers to the intricate science of airflow and particle dynamics. We’ll explore different mask types, materials, and designs, examining their effectiveness in trapping airborne particles and ultimately minimizing the transmission of respiratory infections.
Get ready to uncover the science behind your mask!
From the fundamental principles of droplet transmission to the practical considerations of mask fit and design, we’ll dissect the science behind mask effectiveness. We’ll also examine the scientific evidence supporting their use in public health contexts. Prepare to be amazed by the intricate details of this important topic.
Basic Mechanisms of Mask Functioning
Masks play a crucial role in mitigating the spread of respiratory droplets, which carry viruses and bacteria. Understanding how masks work, and the differences between various types, is essential for informed choices about personal protection. Different materials and designs offer varying degrees of protection, and understanding these mechanisms is key to making the most effective choices.
Respiratory Droplet Prevention
Masks primarily function by creating a physical barrier that intercepts respiratory droplets expelled during coughing, sneezing, or talking. These droplets, ranging in size from tiny aerosols to larger particles, carry infectious agents. The barrier effect of a mask prevents these droplets from traveling through the air and potentially infecting others. This barrier effect is directly related to the mask’s material, structure, and filtration efficiency.
Mask Types and Physical Barriers
Different mask types employ varying physical barriers to trap airborne particles. Cloth masks, the simplest type, rely on a woven fabric structure to catch larger droplets. Surgical masks, more advanced, often incorporate a melt-blown polypropylene layer to filter smaller particles. N95 respirators are designed for higher filtration efficiency, typically using a multi-layered structure with specialized materials to capture even smaller particles.
Material Effectiveness and Filtration
The effectiveness of a mask depends significantly on the material’s ability to trap particles. For example, tightly woven fabrics, like cotton, are more effective at catching larger droplets than loosely woven ones. Melt-blown polypropylene, a common material in surgical masks, is known for its high filtration efficiency due to its unique fibrous structure that creates a dense network capable of intercepting a wide range of particle sizes.
Specialized filters used in N95 respirators often include activated carbon or other substances to remove specific contaminants.
Particle Size and Filtration Efficiency, The simple science behind why masks work
The science behind mask filtration is directly linked to particle size. Masks are designed to capture particles larger than the pores in the mask material. The smaller the particle size, the more challenging it is to trap them effectively. N95 respirators, for instance, are designed to filter out particles as small as 0.3 micrometers, significantly improving their filtration efficiency.
This difference in particle size filtering capability explains why different mask types have varying levels of protection.
Mask Comparison Table
Mask Type | Material | Filtration Efficiency | Breathability Rating |
---|---|---|---|
Cloth Mask | Cotton, linen, silk | Low (typically less than 50%) | High |
Surgical Mask | Melt-blown polypropylene, non-woven fabric | Medium (typically 60-95%) | Medium |
N95 Respirator | Non-woven fabric, melt-blown polypropylene | High (typically >95%) | Low |
Particle Dynamics and Droplet Transmission
Respiratory droplets, the tiny particles expelled during coughs, sneezes, and even normal breathing, play a crucial role in the transmission of respiratory infections. Understanding their characteristics and how they interact with the environment is essential to comprehending how infections spread and how masks can mitigate this transmission. This section delves into the dynamics of droplet transmission and the impact of masks on this process.
Respiratory Droplet Characteristics
Respiratory droplets exhibit a wide range of sizes and shapes, influencing their behavior in the air. These droplets originate from the respiratory tract and vary considerably in size, ranging from microscopic to relatively large. Their size and shape greatly impact their trajectory and how they interact with the surrounding environment. Droplet size is a critical factor in determining their lifespan and potential for transmission.
Understanding the simple science behind masks is fascinating. They work by filtering out airborne particles, which is crucial for respiratory health. This principle, however, is just one piece of a larger puzzle when considering how we can improve outcomes for children’s health, like can more be done to reduce children’s asthma attacks. Ultimately, a deeper understanding of the science behind respiratory health, from simple mask use to complex asthma management, can lead to better outcomes for all.
Small droplets can remain suspended in the air for extended periods, increasing the risk of inhalation by others. Larger droplets tend to fall more quickly, but can still pose a risk of transmission through close contact. The shape of droplets also contributes to their behavior; irregular shapes can affect their stability and the rate at which they fall.
Influence on Transmission
The size, shape, and velocity of respiratory droplets directly affect their ability to travel and transmit infection. Smaller droplets, often referred to as aerosols, can remain suspended in the air for longer periods, increasing the distance over which transmission is possible. Larger droplets, typically referred to as larger droplets, fall more quickly, but can still pose a risk of transmission through close contact, particularly when the person is within a few feet of the source.
The velocity at which these droplets are expelled during an activity like coughing or sneezing also impacts their range and trajectory. The force behind the expulsion affects how far and in what direction the droplets travel. These characteristics influence the likelihood of an individual inhaling or coming into contact with these droplets, thereby increasing the risk of infection.
Airflow and Droplet Dispersion
Airflow plays a crucial role in the dispersion of respiratory droplets. Air currents, whether from ventilation systems, wind, or other factors, can carry droplets further, increasing the potential for transmission. Air movement patterns can affect how droplets are dispersed, and how far they travel. The force of the airflow interacts with the velocity and size of the droplets, causing them to be carried in different directions and distances.
In environments with poor ventilation, droplets can accumulate, increasing the risk of infection. Understanding these factors is critical to developing strategies for preventing transmission in various settings.
Mask Influence on Droplet Transmission
Masks act as a barrier to the spread of respiratory droplets by intercepting them before they can travel significant distances. The material and design of the mask dictate its effectiveness. Masks can significantly reduce the number of droplets expelled into the air. This is achieved by trapping the droplets and aerosols that are produced when speaking, coughing, or sneezing.
Ever wondered how masks actually work? It’s all about simple physics – blocking the spread of airborne particles. This is crucial, as similar principles can help understand other health issues. For example, people with Parkinson’s disease can experience various eye problems, such as dry eyes or difficulty focusing eye problems common in people with parkinsons disease , which might be related to issues in nerve function.
Ultimately, understanding the basic science behind masks is key to comprehending health concerns in general.
Masks are particularly effective at blocking larger droplets, which typically fall more quickly. The effectiveness of a mask depends on factors such as its material, fit, and the particle size of the droplets being expelled. This is vital in controlling the spread of infectious diseases in crowded environments.
Droplet Transmission Flowchart
[Start] --> [Cough/Sneeze/Breathing] --> [Droplet Formation] --> [Droplet Dispersion] (influenced by airflow, size, velocity) --> [Transmission Risk] (depending on distance, exposure) --> [Mask Intervention] (intercepts droplets) --> [Reduced Transmission] --> [End]
Particle Size, Mask Type, and Filtration Efficiency
Particle Size (µm) | Mask Type | Filtration Efficiency (%) |
---|---|---|
<0.1 | N95 Respirator | 95 |
0.1-1 | Surgical Mask | 60-70 |
>1 | Cloth Mask | 20-40 |
The table above illustrates the general relationship between particle size, mask type, and filtration efficiency. N95 respirators are designed to filter out smaller particles, while surgical masks are effective against larger droplets.
Cloth masks offer the least filtration. The filtration efficiency is a measure of the mask’s ability to block particles of a particular size. It’s important to note that these are general estimates, and the actual efficiency can vary based on specific mask characteristics and usage.
Mask Design and Material Properties
The effectiveness of a mask in filtering airborne particles hinges significantly on its design and the properties of the materials used. A well-designed mask, with consideration for both filtration efficiency and user comfort, is crucial for its practical application. Different materials and their inherent properties play a vital role in achieving optimal particle filtration and breathability.
Understanding the interplay between mask design features, material properties, and filtration efficiency allows for the creation of more effective and user-friendly personal protective equipment (PPE). Optimizing these factors is critical in maximizing the protective capabilities of masks, ensuring they provide the required level of protection against various airborne contaminants.
Importance of Mask Design Features
Mask design features significantly impact filtration efficiency. Properly designed masks utilize strategically placed layers and structural components to maximize the interception of particles. This includes the shape of the mask, the fit to the face, and the configuration of the material layers. A well-fitting mask ensures a tight seal around the nose and mouth, minimizing the leakage of particles and maximizing filtration.
Different Mask Materials and Their Properties
Various materials are used in mask construction, each with unique properties affecting their filtration performance. Common materials include woven fabrics, non-woven fabrics, and meltblown materials.
- Woven Fabrics: Woven fabrics, like cotton, are relatively inexpensive but often have lower filtration efficiency. Their filtration performance depends heavily on the tightness of the weave. A tighter weave typically results in better filtration, but breathability can be compromised.
- Non-woven Fabrics: Non-woven fabrics, often made from synthetic fibers like polypropylene, offer a higher filtration efficiency compared to woven fabrics. Their structure, characterized by randomly arranged fibers, traps particles effectively. Different non-woven materials vary in their porosity, impacting both filtration and breathability. Meltblown fabrics, a specific type of non-woven fabric, are known for their high filtration efficiency due to their fine and dense structure.
- Meltblown Materials: Meltblown materials are known for their exceptional filtration efficiency. The fibers are incredibly fine and entangled, creating a dense barrier that effectively traps airborne particles. However, meltblown materials are often less breathable than other types of fabrics. They are often used as the inner layer of a multi-layered mask.
Influence of Material Properties on Filtration
The porosity of a material directly influences its filtration ability. A material with low porosity, meaning fewer tiny openings, will effectively trap more particles. Conversely, high porosity allows particles to pass through. Breathability, on the other hand, is inversely related to porosity. A breathable mask allows for easier airflow through the material.
The strength of the material is also important. A strong material can withstand repeated use and maintain its shape, preventing deformation that can compromise the mask’s seal and filtration effectiveness.
Comparison of Weaving Patterns and Designs
Different weaving patterns in fabrics influence the filtration efficiency of masks. A tightly woven pattern generally offers better filtration than a loosely woven pattern. The precise design of the mask’s layers can also significantly affect the overall filtration capability. For instance, using multiple layers of different materials with varying porosities can create a layered filtration system, trapping a wider range of particles.
Understanding the simple science behind masks is fascinating. It’s all about blocking the spread of tiny airborne particles. This concept, crucial for public health, surprisingly also relates to the mental health challenges faced by college freshmen. Navigating the new social landscape and adjusting to a different environment can be tough on mental wellbeing, college frosh mental health issues are often overlooked.
Luckily, just like masks filter the air, strong support systems can filter out stress and anxiety. Ultimately, both masks and support systems are vital for a healthier environment.
Comparison of Different Mask Materials
Material | Porosity | Breathability | Filtration Efficiency |
---|---|---|---|
Cotton | High | High | Low |
Non-woven polypropylene | Medium | Medium | Medium to High |
Meltblown polypropylene | Low | Low | High |
N95 respirator | Very Low | Low | Very High |
Airflow and Mask Fit
Masks work by obstructing the flow of airborne particles. However, the effectiveness of this obstruction depends critically on the mask’s fit and the airflow around it. A poorly fitting mask allows particles to bypass the filtering material, significantly reducing its protective capabilities. Proper fit, on the other hand, creates a seal that effectively traps particles, maximizing protection.
Understanding airflow and its interaction with the mask is essential for optimizing its filtration efficiency. This knowledge allows us to design and use masks more effectively, minimizing the risk of transmission. A deeper dive into the interplay between airflow, mask fit, and facial features reveals critical details that contribute to a comprehensive understanding of mask functionality.
Impact of Airflow on Mask Filtration Efficiency
Airflow plays a pivotal role in a mask’s filtration performance. The mask’s ability to filter out particles depends on the air velocity and the pressure differential across the mask. High airflow velocities can cause particles to bypass the filter material, reducing the mask’s filtration efficiency. Conversely, a well-designed mask can use airflow to its advantage, ensuring particles are captured effectively.
Proper Mask Fit and Minimizing Leakage
A proper mask fit is crucial for maximizing protection. A good fit creates a seal against the face, preventing leakage of air and particles around the mask’s edges. This seal minimizes the risk of exposure to contaminated aerosols and droplets. Leakage at the edges allows particles to pass through the mask, rendering it ineffective. Consequently, ensuring a snug and secure fit is essential for achieving optimal protection.
Mask-Fitting Methods and Effectiveness
Various mask-fitting methods exist, each with its own level of effectiveness. Correct placement is critical, and methods like using a nose clip for a tighter seal and ensuring the mask covers the mouth and nose are essential steps.
- Correct placement and seal: Ensuring the mask conforms snugly to the face, with minimal gaps around the edges, is essential. The mask should fit close to the face without being uncomfortable. This minimizes leakage and maximizes filtration efficiency.
- Nose clip use: Nose clips, if present, should be properly adjusted to create a tight seal against the nose bridge. This helps prevent air leakage through the nasal passages, improving filtration efficiency.
- Adjusting mask straps: Adjusting straps to achieve a secure fit is crucial. Straps that are too loose will allow air and particles to leak around the edges, whereas straps that are too tight may cause discomfort or irritation. A proper fit ensures a secure and comfortable experience, while minimizing leakage and maximizing protection.
Impact of Facial Features on Mask Fit
Facial features like the shape of the nose, the curvature of the cheeks, and the jawline can influence how well a mask fits. Masks designed to accommodate various facial shapes are more likely to provide a good fit for a broader range of users. Individual differences in facial features can impact the seal and effectiveness of a mask.
Understanding these influences is essential for designing masks that effectively protect a wide range of individuals.
Effect of Mask Fit on Filtration Efficiency
Mask Fit | Filtration Efficiency | Leakage Points |
---|---|---|
Excellent Fit (minimal gaps) | High (95%+) | Minimal or no leakage |
Good Fit (some gaps) | Moderate (70-90%) | Minor leakage around edges |
Poor Fit (significant gaps) | Low (<70%) | Significant leakage around edges, nose, chin |
This table highlights the direct relationship between mask fit and filtration efficiency. A tighter fit generally leads to higher filtration efficiency, while significant gaps compromise the mask’s ability to prevent particle transmission.
Scientific Evidence and Research
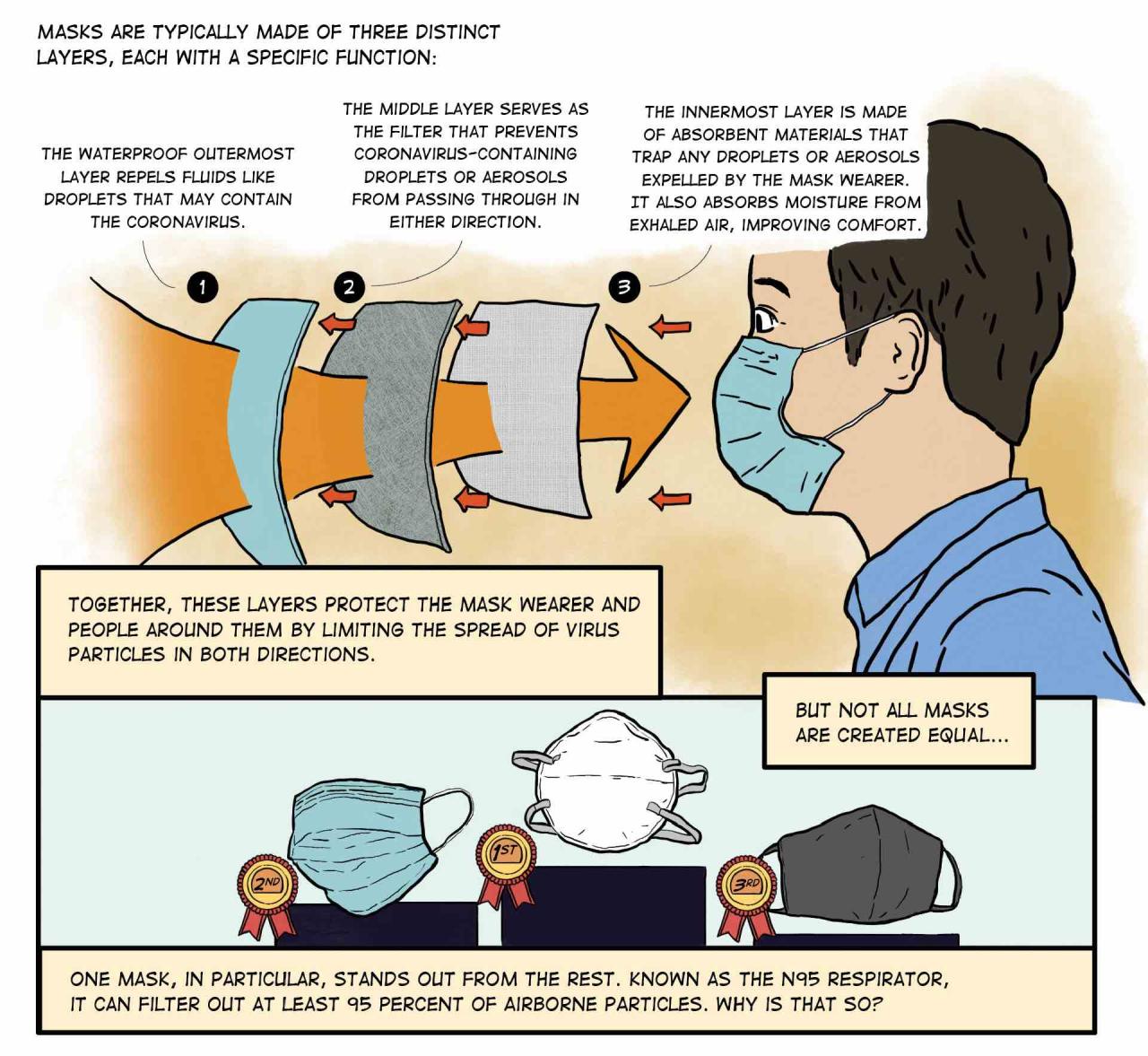
Understanding the effectiveness of masks requires rigorous scientific investigation. This involves carefully designed studies that measure mask performance under controlled conditions, and the results must be evaluated within the context of the specific study design. Different methodologies yield varying degrees of confidence in the conclusions. Crucially, these studies contribute to public health recommendations by providing evidence-based support for mask use.
Research Methodologies for Evaluating Mask Effectiveness
Various methodologies are employed to assess mask performance. These methodologies include laboratory testing, field studies, and randomized controlled trials. Laboratory testing, often using standardized aerosol or particle generators, allows researchers to quantify the filtration efficiency of different mask types under controlled conditions. Field studies, observing mask use in real-world settings, provide insights into how masks affect transmission in diverse populations.
Randomized controlled trials, often comparing mask use to no mask use, provide stronger evidence of causality. Each approach has its strengths and limitations, influencing the degree of confidence that can be placed in the conclusions.
Different Study Designs to Measure Mask Performance
Different study designs are utilized to assess mask effectiveness, each with its strengths and limitations. Observational studies, where researchers track mask use and infection rates in a population, can identify correlations but cannot definitively prove causation. Interventional studies, where researchers actively assign participants to mask or no-mask groups, are considered stronger evidence of causality, especially randomized controlled trials.
The specific design employed dictates the degree of certainty that can be placed on the findings.
Results of Scientific Studies on Mask Use and its Effect on Respiratory Infections
Numerous studies have investigated the impact of mask use on the spread of respiratory infections. These studies demonstrate that mask-wearing can significantly reduce the transmission of respiratory droplets and aerosols, especially in enclosed spaces. Findings show varying degrees of effectiveness across different mask types, with higher filtration efficiency masks generally correlating with lower transmission rates.
Key Findings from Peer-Reviewed Research
Key findings from peer-reviewed research consistently highlight the effectiveness of mask-wearing in reducing the spread of respiratory infections. Studies have shown a correlation between mask use and a decrease in the incidence of infections, particularly in healthcare settings and during outbreaks. The strength of this correlation is influenced by the specific design and execution of the study. These findings have led to the widespread adoption of mask mandates in public health settings and have provided strong support for the use of masks in various public settings.
How the Scientific Community Supports Mask Use for Public Health
The scientific community has used the data from various studies to support the use of masks for public health. Peer-reviewed publications, systematic reviews, and meta-analyses synthesize the evidence, providing a comprehensive understanding of the impact of masks on transmission. These comprehensive evaluations influence public health guidelines and recommendations, contributing to a more informed approach to mitigating respiratory infection outbreaks.
This process ensures that public health measures are based on robust scientific evidence.
Illustrative Examples of Mask Functionality: The Simple Science Behind Why Masks Work
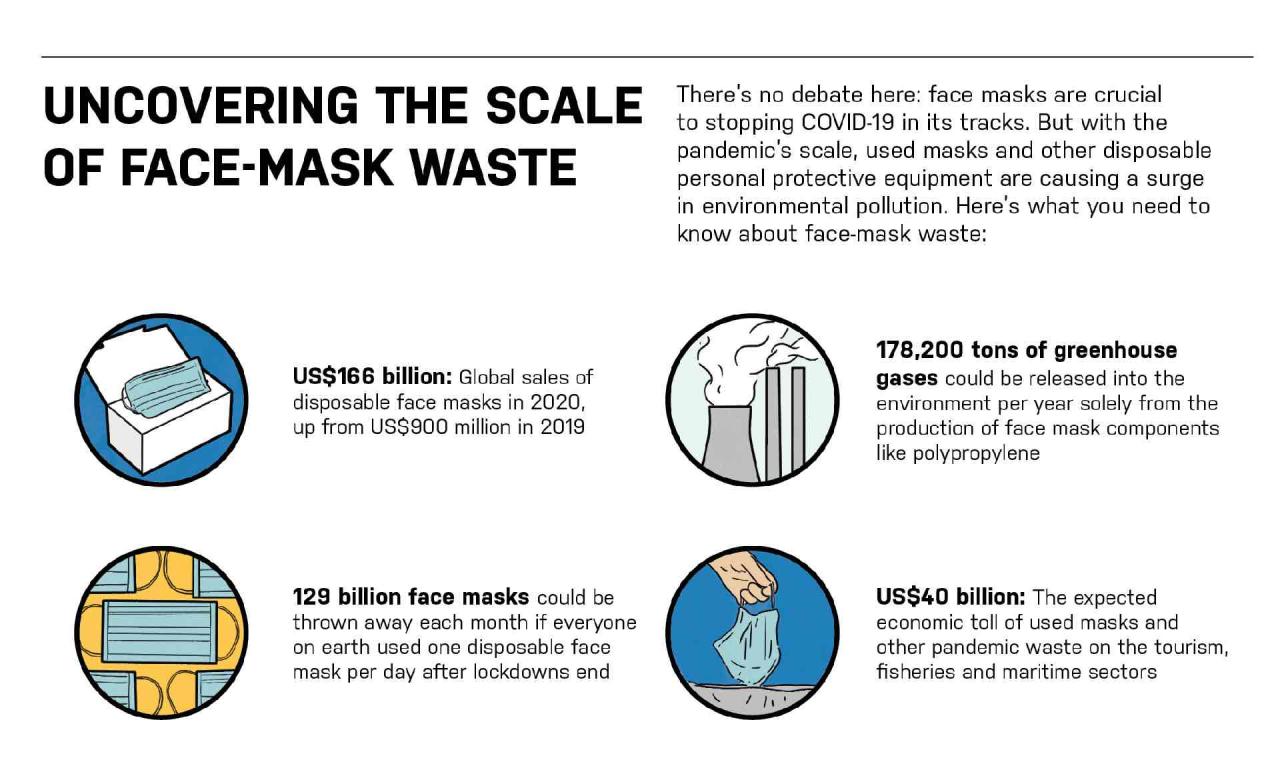
Masks play a crucial role in mitigating the spread of respiratory droplets and aerosols. Understanding how different mask types function and their limitations is vital for effective preventative measures. This section provides detailed examples of mask functionality, focusing on filtration, fit, and overall effectiveness.
Different Mask Types and Their Functionalities
Various mask types offer different levels of protection, depending on their design and materials. A basic understanding of these differences is important for informed choice.
- Surgical Masks: These masks, often used in healthcare settings, are designed to prevent the wearer from spreading respiratory droplets. They typically feature a loose-fitting structure with a single layer of non-woven material. The outer layer acts as a barrier to catch large droplets expelled by the wearer, while the inner layer prevents direct contact of the wearer with the potentially contaminated exterior layer.
These masks are effective at preventing the wearer from spreading large droplets, but may not be as effective at preventing the wearer from inhaling smaller airborne particles.
- N95 Respirators: These respirators are designed for higher filtration efficiency, providing protection against a broader range of airborne particles, including smaller droplets and aerosols. The N95 designation indicates that the mask filters out at least 95% of airborne particles. They have a tighter fit, creating a seal around the nose and mouth, preventing particles from entering. This tighter fit can be uncomfortable for some individuals, and requires proper training for effective use.
The use of N95 masks can be crucial in environments with high particle counts, like hospitals, or for individuals with underlying respiratory conditions.
- Cloth Masks: These masks are typically made of fabric and are often used in everyday settings. Their effectiveness varies significantly depending on the material and construction. Some cloth masks can offer a degree of protection against large droplets, while others may offer little or no protection. They are generally more comfortable to wear than N95 masks but less protective.
The material composition plays a significant role in filtration, with denser materials providing more filtration than lighter ones.
Filtration Process in Masks
Masks work by filtering out particles from the air that the wearer breathes. The filtration process is a multi-step mechanism.
- Particle Encounter: The mask intercepts airborne particles, such as droplets and aerosols, as the wearer breathes. The size, shape, and density of these particles will affect how effectively they are captured.
- Entrapment: The mask’s material structure traps these particles. This can happen through physical interception, where the fibers of the material catch the particles, or through electrostatic forces, where the particles are attracted to the fibers. The specific mechanism depends on the material composition of the mask.
- Removal from Airstream: The trapped particles are effectively removed from the air that the wearer breathes, preventing them from entering the respiratory system.
Filtration Efficiency Comparison
Different masks have varying filtration efficiencies, impacting their protective capabilities. This difference is directly related to the mask material and design.
Mask Type | Typical Filtration Efficiency (%) | Description |
---|---|---|
Surgical Mask | Around 50-70% | Provides a basic level of protection against large droplets. |
N95 Respirator | >95% | Designed for high filtration efficiency against a broad range of particles, including smaller droplets and aerosols. |
Cloth Mask (Dense Fabric) | 20-80% (Variable) | The effectiveness depends heavily on the fabric type and construction. |
Effect of Mask Fit on Droplet Spread Prevention
A proper mask fit is crucial for effective protection. An improper fit allows leakage, significantly reducing the mask’s ability to prevent the wearer from inhaling particles or spreading droplets.
Visual Representation of Mask Fit: The diagram illustrates the difference between a properly fitted mask (left) and a poorly fitted mask (right). The properly fitted mask creates a tight seal around the nose and mouth, preventing leakage and ensuring efficient filtration. The poorly fitted mask allows air and particles to leak through, significantly reducing the mask’s protective capabilities.
Closing Summary
In conclusion, the science behind mask effectiveness is multi-faceted, encompassing particle dynamics, mask design, and airflow. Understanding the different mask types, their materials, and filtration efficiency is crucial for informed choices. We’ve covered the scientific evidence, highlighting the importance of proper mask fit and the overall impact of mask use on respiratory infection transmission. Hopefully, this detailed exploration of the science behind why masks work empowers you to make well-informed decisions about their use.